Astrophysics/cosmology research
Study of neutron stars
Professors Edward Cackett, Renee Ludlam
Neutron stars are fascinating objects. They are formed in a supernova explosion at the end of a star's life: what is left after the explosion is a tiny, incredibly dense star.
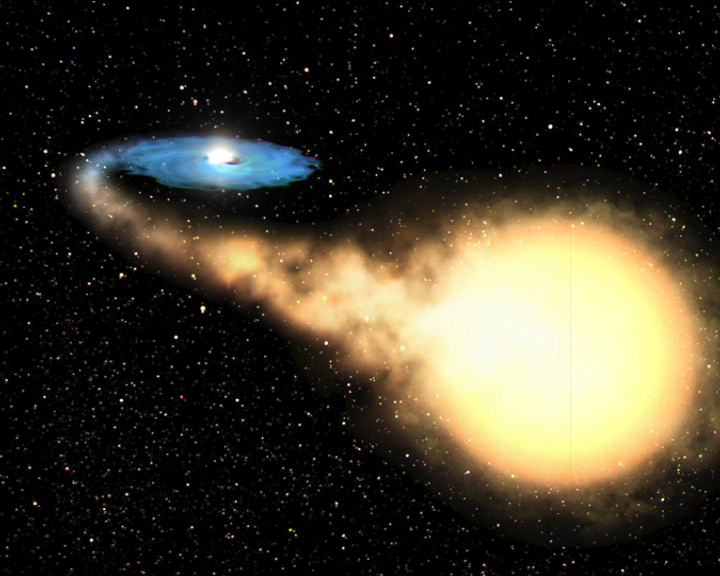
They have a mass a little more than that of our sun, yet are crammed into a sphere only about 20-30 km across. This makes the very centers of neutron stars more dense than atomic nuclei! On Earth, we cannot reproduce those conditions experimentally, which makes neutron stars a unique astrophysical laboratory to study the densest observable material in the universe. What neutron stars are made of is a vital question that underpins our knowledge of the way fundamental physics works how does matter behave when it is compressed to such extreme densities?
Drs. Cackett and Ludlam use world-leading X-ray satellites to observe neutron stars in binary systems where a star similar to the sun and a neutron star orbit each other. In such systems, the gravity of the neutron star can pull matter from the companion, which then spirals down onto the neutron star forming a hot disk of gas (an 'accretion disk') around it. They study this accretion process and its effects on the neutron star in order to learn about the nature of these superdense objects.
For more information about this research, visit the Chandra X-Ray Observatory.
Study of black holes
Professors Edward Cackett, Renee Ludlam
Black holes are objects so dense that even light cannot escape their gravitational pull. They can come in several different masses - stellar-mass black holes have masses that are typically 10 tens the mass of our Sun, and, like neutron stars, are formed in supernovae explosions at the end of a massive star's life. Supermassive black holes, on the other hand, have masses typically between a million and a billion times the mass of the Sun and every major galaxy in the universe, including our own Milky Way, seems to have one at their center. Astronomers still don't know exactly how they form.

Dr. Cackett uses multiwavelength observations to investigate how material falls onto black holes. Since the angular size of most black holes is far too small to be imaged by current technologies, indirect techniques must be used. Dr. Cackett uses a technique called reverberation mapping that measures echoes of light to try and reconstruct the size of structure of the gas falling into black holes. He uses a range of facilities includes NASA's Hubble Space Telescope, the Neil Gehrels Swift Observatory, the NICER X-ray mission as well as Wayne State's own Dan Zowada Memorial Observatory. In January 2019, Cackett co-authored work featured on the front cover of prestigious journal Nature - you can read a brief description here.
Theoretical black holes can be fully described by three properties: mass, charge, and spin (a measure of their angular momentum). Since astrophysical black holes are thought to have no charge, this leaves two remaining quantities (mass and spin). The accretion disks around black holes are able to reprocess energetic photons and re-emit intrinsically narrow emission lines that are broadened due to Doppler, General, and Special relativistic effects from being within the strong gravity regime near the black hole. The ability to measure the broadening of these lines thereby allows for estimates of the spin of the black hole since these effects become stronger as a function of proximity to and rotation rate of the compact object. Dr. Ludlam uses X-ray observatories to detect broadened emission lines that can be used to determine black hole spins.
Study of active galactic nuclei
Professor Tonima Tasnim Ananna
Supermassive black holes are believed to reside at the center of nearly all large galaxies. Space objects devour galactic gas, dust, and stars. They can even become heavier than some small galaxies.
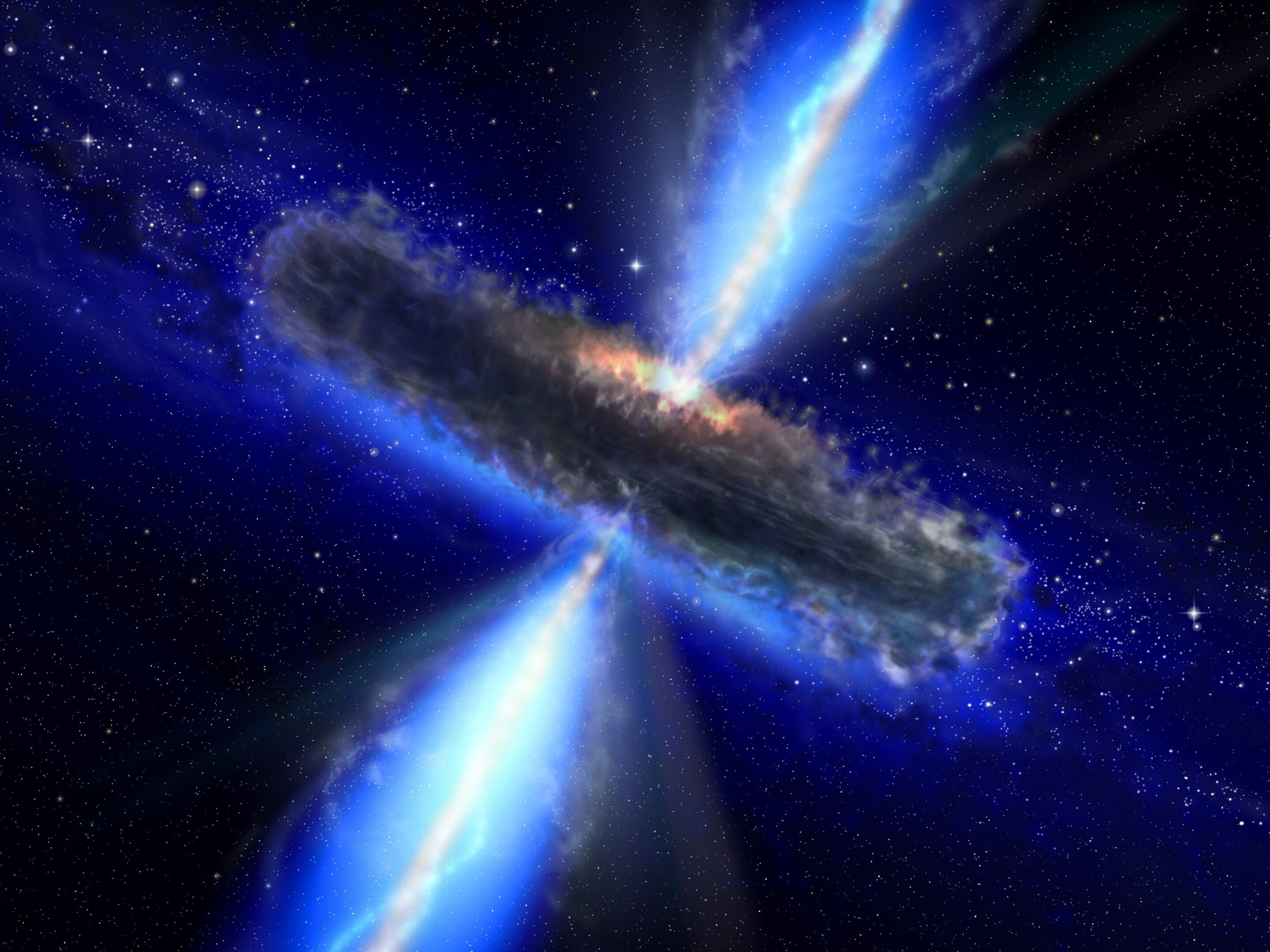
By knowing how fast a black hole is feeding, its mass, and the amount of radiation nearby, researchers can determine when some black holes underwent their biggest growth spurts. That information, in turn, can tell them about the history of the universe.
Light coming from near supermassive black holes can have different colors. They can also vary in brightness and spectral signatures. Until recently, researchers believed that the differences depended on viewing angle and how much a black hole was obscured by its "torus," a doughnut-shaped ring of gas and dust that usually surrounds active galactic nuclei (AGN).
Dr. Ananna develops novel computational techniques to assess the effect of obscuring matter on the observed properties of black holes. Her most recent study shows that the amount of dust and gas surrounding a supermassive black hole is directly related to how actively it is growing. When a black hole feeds at a high rate, the energy blows away dust and gas. As a result, it is more likely to be unobscured and appear brighter. The research provides some of the strongest evidence yet that there are fundamental differences between supermassive black holes with different light signatures, and that these differences cannot be explained only by whether the observation is taking place through or around an AGN's torus. The study definitively shows the need to revise the prevailing theory of AGN, which characterizes obscured and unobscured AGN as similar, despite appearing different due to viewing angle.
Dr. Ananna's work was featured in Science News' top 10 scientists to watch in 2020.
Dan Zowada Memorial Observatory
The Dan Zowada Memorial Observatory consists of a state-of-the-art 20-inch PlaneWave telescope with a PlaneWave L-500 direct-drive mount on Palomar Mountain in southern California at an elevation of 5,000 ft. Relocated from the high desert of Rodeo, NM, the robotically-controlled remote observatory supports key research projects requiring nightly imaging of galaxies.